Research
Visual cortical dysfunction in Parkinson Disease
Non-motor symptoms are increasingly recognized as important contributors to overall disability and quality of life in patients with Parkinson disease (PD) and dementia with Lewy bodies (DLB). Cognitive impairment and hallucinations are defining features of DLB and are also common in PD. In both DLB and PD, impairments in visuospatial and visual perceptual functions are prominent. These functional impairments correlate with neuroimaging data showing early hypometabolism of the posterior parietal and occipital lobes and, later, cortical thinning and atrophy in these same structures. Together, these findings suggest the visual cortical system is an important site of pathology in these disorders. Accumulation and aggregation of the protein a-synuclein is hypothesized to play a central role in the pathogenesis of PD and DLB. Although a-synuclein has well recognized roles in synaptic transmission, including vesicle fusion and neurotransmitter release, the effects of a-synuclein pathology on cortical circuit function are not well studied.
To date, there remain significant gaps in our knowledge of how a-synuclein pathology leads to cortical dysfunction. Hypotheses include pre-synaptic denervation due to nigrostriatal degeneration, a-synuclein mediated impairment of cortical neuron activity, and direct cytotoxicity to cortical neurons. To begin to address these possibilities, we are using longitudinal in vivo two-photon calcium imaging in mice to directly record changes in neuronal ensemble activity of visual cortical circuits throughout the progression of a-synuclein pathology. Our approach allows us to simultaneously image the formation and progression of a-synuclein inclusions and activity of individual cortical neurons longitudinally over time. We also plan to investigate the differential effects of distinct a-syn strains isolated from patients afflicted with synucleinopathies (PD/DLB or multiple system atrophy) on cortical circuit dysfunction.
These studies will fill important gaps in our knowledge of the mechanisms underlying cognitive symptoms in synucleinopathies that we hope will inform future therapeutic strategies for PD and DLB.
Pictured: Staining of α-synuclein pathology.
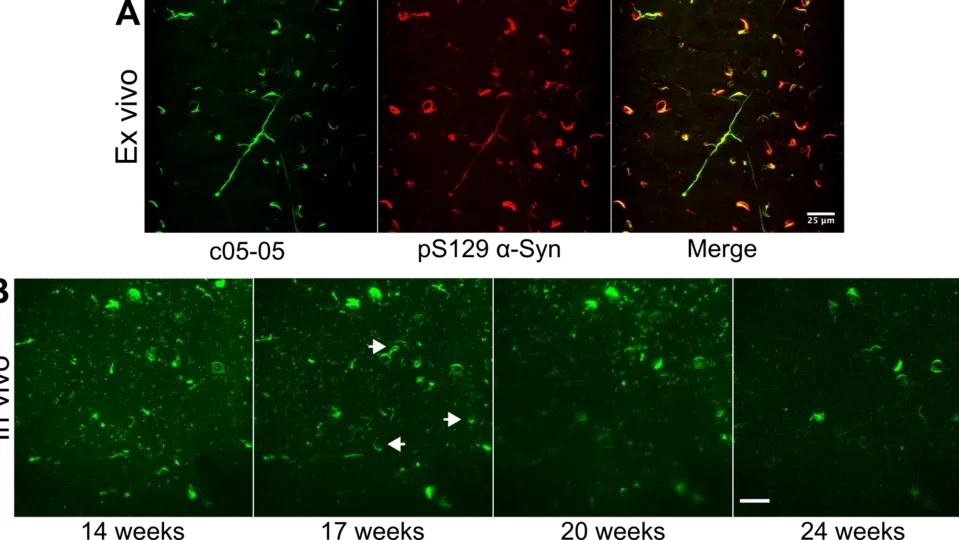
Functional Circuit Remapping After Stroke
Following ischemic stroke, many patients exhibit partial spontaneous recovery, suggesting that the brain has endogenous mechanisms to recover lost functions. There has been considerable interest in harnessing this process of remapping for therapeutic purposes, especially after the failure of neuroprotective strategies in stroke.
However, evidence for remapping from neuroimaging and neurophysiologic studies on human stroke patients has been contradictory and difficult to interpret, in part due to the stochastic nature of human stroke. Thus, how specific changes in neuronal circuits mediate improvement in function and recovery after stroke remains a major gap in our understanding of remapping.
Traditional approaches to studying remapping in humans (e.g., fMRI, PET) lack the temporal and spatial resolution to precisely address this question. At the same time, more recent techniques for assessing remapping with single cell resolution have yet to be rigorously applied to experimental mouse models of stroke.
We are utilizing a multi-modal imaging approach in mice, including two-photon calcium imaging, genetic labeling of active neurons, and chemogenetics to define both the local and global changes in neural circuits that mediate functional remapping and recovery after stroke. These studies will fill essential gaps in our knowledge and are highly relevant for current and future translational studies targeted to improve recovery after stroke.
Pictured: Models of L2/3 whisker somatosensory circuit changes post-stroke.
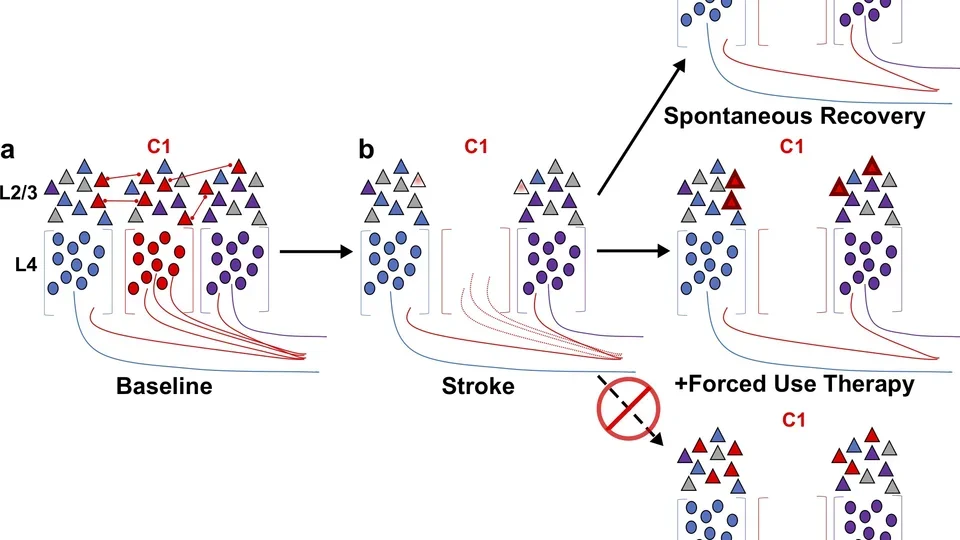
Brain Plasticity After Stroke
A high-level overview of plasticity of the cortex during stroke recovery.